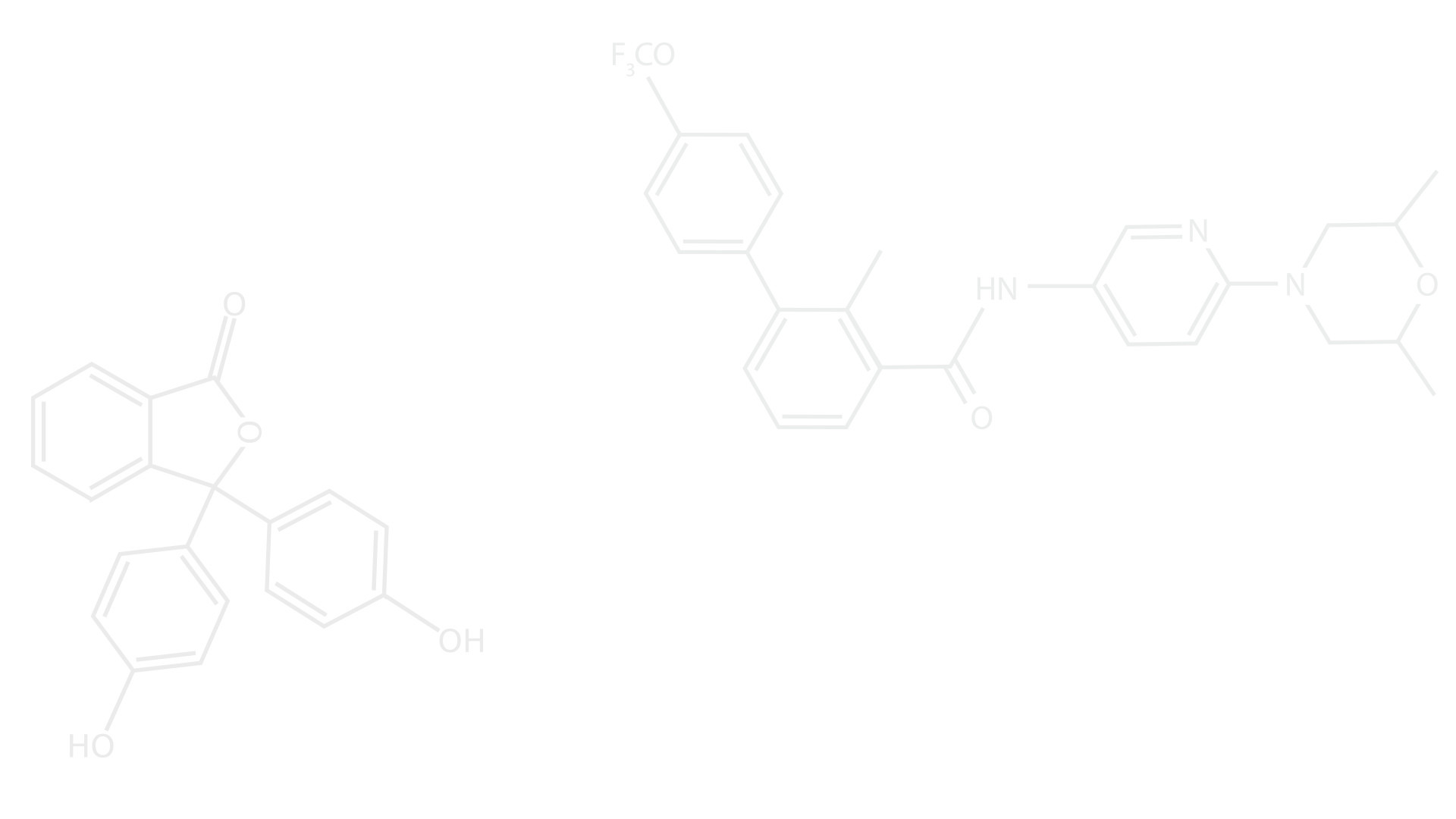
Research Highlights
Here are some highlights of key research from our group. For a more detailed overview, please refer to our publications.
1. Self-organization of Biomacromolecules
​
This research aims to explore the complex processes of self-assembly and phase transitions in biomacromolecules, with a particular emphasis on intrinsically disordered proteins (IDPs). IDPs are characterized by a unique confluence of various attributes, including electrostatic and hydrophobic interactions among residues, chemical sequence, and the degree of blockiness in specific types of sequences, such as positively charged, negatively charged, hydrogen-bonded, and hydrophobic segments.
The self-organization of these proteins into membraneless organelles, droplets, and fibrillar aggregates is crucial to numerous biological functions and diseases. Utilizing state-of-the-art coarse-grained computational models and simulations, we investigate the kinetics and dynamics of biocondensate formation and examine the conditions under which IDPs in biocondensates transition from disordered states to structured fibrils. This work holds significant potential for developing new approaches for controlling biomolecular organization at the nanoscale and anti-aggregation therapies for neurodegenerative diseases.
Our research has revealed novel mechanisms of microphase separation in IDPs, challenging the conventional view of simple liquid-liquid phase separation in these systems. We aim to extend this understanding by integrating the influence of nonequilibrium forces and environmental factors on IDP aggregation.
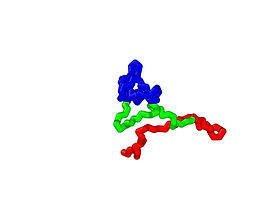

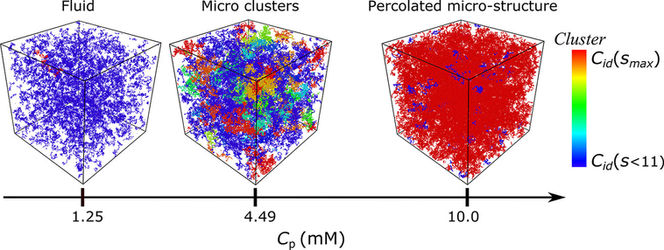
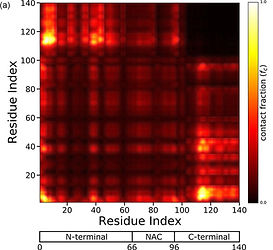
2. Transport and Assembly of Active Materials
Another research focus in our group is on the transport phenomena and self-assembly mechanisms in active materials, which include systems of self-propelled/active colloids and polymers. These systems exhibit behaviors far from equilibrium, providing new opportunities to tune motion and design materials with unique properties that are not achievable under conventional equilibrium conditions.
A central aspect of this work is the development of multiscale computational models that capture the interaction between thermal and active fluctuations, along with hydrodynamic forces. We have advanced the capabilities of Multi-particle Collision Dynamics (MPCD), enabling us to model the distinctive transport behaviors exhibited by self-propelled particles. One of our key findings has been the demonstration of how active forces can manipulate the configuration and morphology of macromolecules.
In collaboration with experimental researchers, we discovered hydrodynamic turbulence in a dispersion of active spinners, which self-assemble from magnetic colloids energized by an alternating magnetic field. Our findings has provided valuable insights into the behavior of active spinner liquids and methods to control the collective dynamics and transport in active colloidal systems. Additionally, we have explored the stationary-state distribution of active Brownian particles (ABP) under confinement and examined bulk pressure in localized regions of the system. Our work revealed the existence of a pressure equation-of-state for spherical ABPs and demonstrated that active stress is not merely a boundary effect, as previously thought, but is rather caused by an instantaneous active momentum transport.

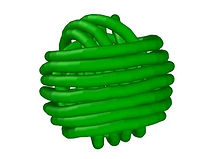

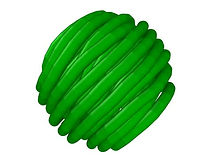


Activity helps in periodically exploring metastable states
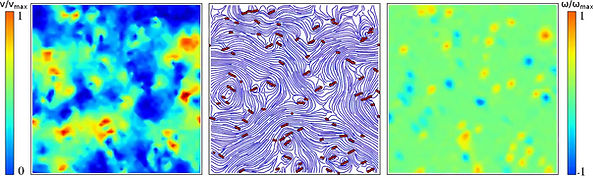
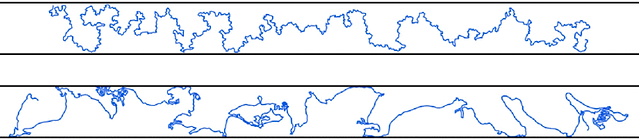
Turbulence in active spinner material
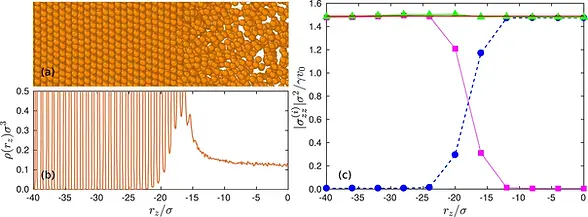
Local stress in inhomogeneous systems of ABPs
Deviation from blob theory for confined polymers with increasing active fluctuations
3. Developing Advanced Simulation Frameworks
Going beyond traditional modeling approaches, we also focus on developing simulation frameworks that leverage modern computational power to capture the full complexity and range of scales observed in experiments. We have integrated distinct computational techniques — continuum modeling and particle-based simulation — that vary in scale, nature, and computational requirements to study the digital fabrication of integral-asymmetric isoporous block copolymer membranes. Continuum modeling offers excellent scalability, enabling the simulation of large-scale physical phenomena, but lacks the fine resolution that a particle-based model can provide. In contrast, particle-based simulations offer high accuracy but come with significantly higher computational costs. To bridge these gaps, we develop hybrid simulation frameworks that combine the strengths of both approaches, achieving both scalability and accuracy. We have tested this coupled modeling strategy by simulating the ordering of a cylinder-forming diblock copolymer melt in response to a moving thermal front, which replicates the Order-Disorder Transition (ODT) during Evaporation-Induced Self-Assembly (EISA).
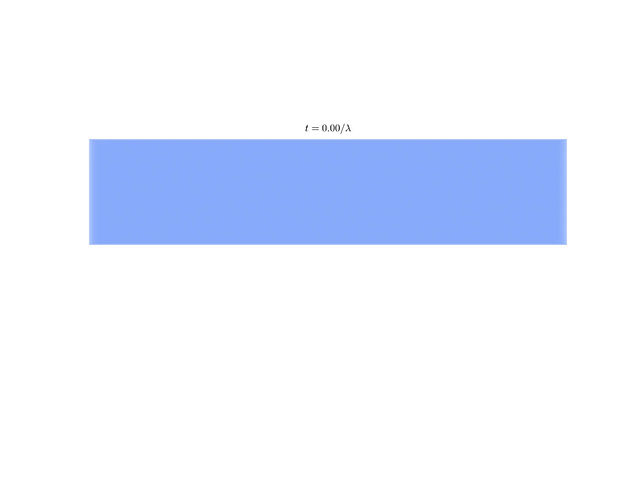
SOMA: particle-based model
UDM: continuum model